Early diet can prevent later disease
May 31, 2006
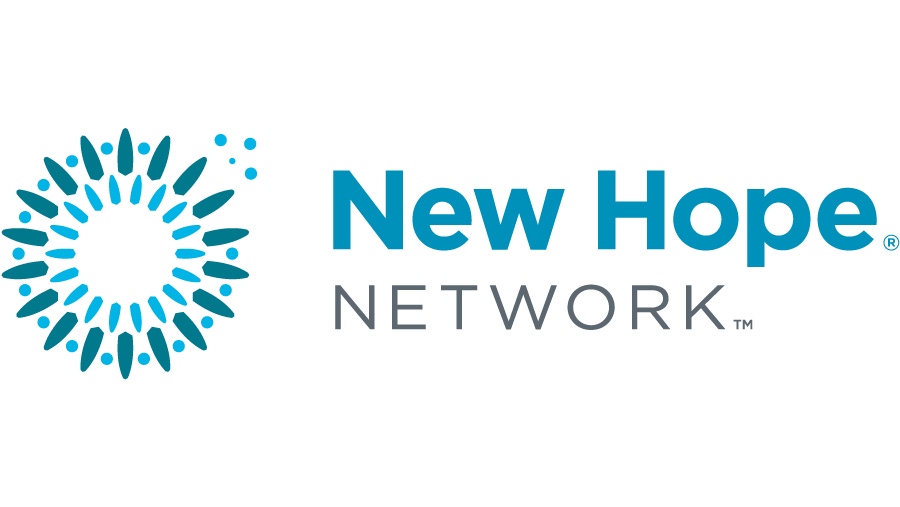
Optimal nutrition of the pregnant and nursing mother, as well as of the young child, is now seen as vital to reduce the risk of adult degenerative diseases. Luise Kalbe, Brigitte Reusens and Claude Remacle explain
Imbalances in maternal nutrition can adversely affect normal foetal growth and development. Children who experience impaired foetal growth are more likely to show poor cognitive development and neurological impairment. Some chronic adult diseases are hypothesized to originate in utero: cardiovascular disease, high blood pressure, obstructive lung disease, diabetes, high cholesterol concentration and renal damage.1
Poor nutrition in foetal and early life may be responsible for this association.2,3 An epidemiological study of a population born in Hertfordshire, UK, for which the birth-weight data were available, found increased death rates from ischaemic heart disease in men with low birth weight and weight at one year of age.4 The concept of foetal origin of adult diseases was then proposed.
Low birth weight is the proxy of poor foetal nutrition. When poor nutrition occurs during foetal development, organ-selective changes in nutrient distribution have to take place so that the growth of some organs will be spared (eg, the brain) to the detriment of other organs (eg, the viscera). This serves the purpose of enhancing postnatal survival under conditions of intermittent and poor nutrition.
It was thus realized that some of the persisting effects of early undernutrition become translated into pathology, and thereby determine chronic diseases in later life. Since then, numerous retrospective and prospective epidemiological studies were undertaken aiming to support this hypothesis of the foetal origin of adult degenerative diseases. The association between low birth weight and coronary heart disease has now been confirmed in Sweden, 5 Finland, 6 United States, 7 and in South India. 8 The same correlation was found between low birth weight or weight at one and the subsequent metabolic syndrome (glucose intolerance, hypertension and raised plasma triglyceride concentration) as well as type 2 diabetes. 9,10
From a continuous supply of substrates through the placenta during pregnancy, to a short period of food withdrawal after birth, followed by intermittent feeding with milk and finally weaning with its transition to solid foods, the infant has to repeatedly adapt to profound changes of nutrition.11 This requires changes in the metabolism of most organs at a period of its life that is characterised by rapid growth and maturation of its entire organism. Different nutrients are needed for each task.
Carbohydrates
The best-studied substrate in human pregnancy is glucose, and there is a direct relationship between maternal blood glucose, foetal glycaemia and size at birth.12 Glucose is indeed the major energy source for the foetus, comprising around 90 per cent of the energy supply. Therefore, maternal carbohydrate metabolism during pregnancy and the source of carbohydrates may be relevant to the optimal supply for the foetus.
Altering the type of carbohydrate consumed (high- vs low-glycaemic sources) changes post-prandial glucose and insulin responses in women, and a consistent change in carbohydrate type eaten during pregnancy influences the rate of foeto-placental growth, maternal weight gain and birth weight. Since changing the type of carbohydrates ingested changes metabolic efficiency and substrate utilisation (glucose vs lipid oxidation), this will favour either insulin resistance or sensitivity and may eventually increase or reduce the risk for later obesity or insulin resistance.13
A high-carbohydrate intake in early pregnancy suppresses placental growth, especially if combined with low dairy protein consumption in late pregnancy.14 The intake of small quantities of animal protein and plenty of carbohydrates or vice-versa in late pregnancy has also been associated with reduced placental size and higher blood pressure in the adult offspring.15
Lipids
Pregnant women have high requirements for lipid-soluble vitamins and polyunsaturated fatty acids. During pregnancy, concentrations of blood lipids and their constituent fatty acids rise sharply.16 All of the n-6 and n-3 fatty acid structure needed by the foetus for normal development must be supplied by the mother and cross the placenta either in the shape of the essential fatty acids linoleic acid (18:2, n-6) or alpha-linolenic acid (18:3, n-3) or their long-chain polyunsaturated fatty acid derivatives such as arachidonic acid (20:4, n-6) or docohexaenoic acid (22:6, n-3).
Arachidonic acid is the main precursor of eicosanoids, prostaglandins and leukotrienes, and is also essential for neonatal growth, whereas docosahexaenoic acid (DHA) plays a role in brain development and visual function.17
A high supply of long-chain n-3 fatty acids may be beneficial to the developing foetus for several reasons: their importance for neural tissue development,18 a lowering of pregnancy-induced hypertension that may induce some obstetric complications,19 or an improvement of the average birth weight without adverse effects on foetal growth or the course of delivery.20,21 However, n-3 fatty acids can have adverse effects, such as higher blood loss on delivery due to suppression of platelet aggregation, and higher perinatal mortality.20
Placental selectivity for alpha-linolenic acid and DHA appears to be relatively unresponsive to changes in the fatty acid mixture in the maternal circulation.22 Excessive consumption of certain long-chain polyunsaturated fatty acids inhibits delta-5- and delta-6-desaturases and leads to declines in arachidonic acid or DHA levels.17 Excess dietary polyunsaturated fatty acids also enhance lipid peroxidation and reduce antioxidant activity.17 Hence, additional studies are needed before recommendations to increase long-chain polyunsaturated fatty acid intake during pregnancy may be made.
On the other hand, it is not clear to which degree the foetus is capable of desaturating and elongating fatty acids, but term and pre-term infants can synthesise long-chain polyunsaturated fatty acids from parental fatty acids.17
Protein and amino acids
Although little is known about the metabolic processes in early foetal organs, important needs for amino acids exist to maintain the intense formation and remodelling of new tissues during embryogenesis and organogenesis. Additional protein is needed during pregnancy to cover the estimated 21g/day deposited in foetal, placental and maternal tissues during the second and third trimesters.23
The recommended increment of protein intake over non-pregnancy values is higher than that of energy during pregnancy. In the first trimester of pregnancy, protein synthesis is similar to that of non-pregnant women and increases respectively by 15 per cent and 25 per cent in the second and the third trimester.24
Folic acid and homocysteine
Since mammals cannot synthesise folic acid, it must be provided by the diet or by intestinal microorganisms. It is essential for the biosynthesis of some amino acids, neurotransmitters, purines and pyrimidines, and hence DNA and RNA. Compromised maternal folate intake or status is associated with several negative pregnancy outcomes including low birth weight, abnormal placenta, spontaneous abortions, or neural tube defects.25,26,27,28 The recommended supplement is a daily dose of 400-600mcg/day.
Such intervention may also influence the programming of cardiovascular disease, since folate modulates the metabolism of homocysteine, which may increase the risk for atherosclerosis.28 Homocysteine is an amino acid involved in several metabolic processes, including the methylation and sulfuration pathways. Blood concentrations of homocysteine are determined by dietary factors such as folic acid and vitamin B12, by altered physiology and by modifications of enzymatic activity due to genetic polymorphism. In normal pregnancy, like those of most of the amino acids, homocysteine concentrations fall.29 Of note, a recent study showed vitamins B6, B12 and folate lowered homocysteine levels in cardiovascular disease patients, but this failed to translate into protection from future CVD events.30
Choline
A low availability of dietary choline during pregnancy alters foetal brain biochemistry and hippocampal development. This induces behavioural changes that persist throughout the lifetime of the offspring. Humans with choline deficiency but with an otherwise balanced diet develop liver damage due to programmed cell death, because de novo synthesis of choline is not enough to make up for this lack of choline.31
In rats, dietary deficiency produced hepatocarcinoma. Female rats were less sensitive to this choline deficiency than males, perhaps because oestrogen enhances their capacity to synthesise choline de novo from S-adenosylmethionine. Pregnant rats were more vulnerable to the lack of choline than males, because maternal stores are depleted due to large-scale transfer of choline to the foetus across the placenta.32 At birth, plasma choline concentrations are considerably higher than in adult human and other mammalian species.32
Postnatal supplementation
Breast milk provides all the nutrients needed to support adequate growth of the term infant during the first 4-6 months of life. It not only provides the recognised nutrients, but also a number of semi-essential nutrients such as enzymes, hormones, oligosaccharides and growth factors that also intervene in infant growth such as intestinal maturation.33,34
A large body of epidemiological evidence supports the association between maternal nutritional deficiency and maternal morbidity, length of pregnancy or foetal growth. The effect of nutritional intervention is also linked to the length of the supplementation and the amount achieved.
Dietary supplementation must be carefully weighed for its potential benefits and risks because what favourably affects the risk for one disease may be detrimental with respect to another.35 Determination of the positive outcome of supplementation studies based only on increased birth weight of the offspring may not be as judicious as might seem and the consequences must be taken into account.
Iron depletion can lead to anaemia in pregnant women, adolescent girls, low-birth-weight infants and in populations in which malaria and parasite-induced blood losses are endemic. Iron depletion can be overcome by iron fortification, but iron supplements frequently fail to restore haemoglobin concentrations to normal during intervention periods with high iron requirements such as pregnancy.
Vitamin A, riboflavin or folic acid can overcome anaemia when added to the iron supplement and administered to pregnant or lactating women, as well as children.36 Iron supplementation during pregnancy not only increases the maternal iron stores during pregnancy and reduces the risk of adverse pregnancy outcomes,36 but it also increases iron stores of the infant and of the mother post-partum.37
Folic acid supplementation is widely carried out during pregnancy in order to reduce the risk of neural tube defects. These defects increase, however, with increasing prepregnancy weight, independent of the mother's folate intake.38 In other words, folate loses its protective effect in overweight and obese mothers.39
Taurine supplementation was never investigated during pregnancy and early life in humans, but some arguments indicate a potential benefit. Taurine, a free amino acid that is not incorporated into proteins, is important for development. Taurine is one of the most abundant free amino acids in human milk.40 Since humans, especially infants, depend on exogen sources of taurine, researchers have suggested that synthetic formulas be supplied with taurine.41,42
Omega-3 and n-6 polyunsaturated fatty acids are essential for the organism, especially during development, and contrary to widespread belief n-6 polyunsaturated fatty acids per se are not detrimental to our health. The important factor seems to be a proper balance between n-6 and n-3 polyunsaturated fatty acids, especially during growth and development.43,44
Unfortunately, the intake of n-3 polyunsaturated fatty acids has decreased with lower fish consumption and increased industrial production of animal feeds rich in n-6 polyunsaturated fatty acids. This has led to meats, eggs and cultured fish rich in n-6 polyunsaturated fatty acids. Even cultivated vegetables contain fewer n-3 polyunsaturated fatty acids than those in the wild.45 This shift in n-6 to n-3 ratio has led to an increase in cardiovascular disease, type 2 diabetes, etc., and supplementation of the diet aimed at improving this ratio has been beneficial in this respect.45
The recommendations for pregnant and lactating mothers are the same as for the rest of the adult population, whereas recommendations for milk formula suggest simultaneous reduction of n-6 and increase of n-3 polyunsaturated fatty acids. A recent study has demonstrated that maternal supplementation with very long-chain n-3 fatty acids coming from cod liver oil during gestation and lactation augments children's IQ at four years of age.46 This beneficial effect was not obtained with n-6 fatty acids.
Others: From recent results of systematic reviews of randomised trials of nutritional interventions during pregnancy, it appears that no specific nutrient supplementation was identified for reducing preterm delivery. However, iron and folate supplementation reduces anaemia and therefore should be included in antenatal care programmes. Calcium supplementation appears promising for women with low calcium intakes who are at high risk for preeclampsia and hypertension. Fish oil and vitamins E and C could prevent preeclampsia.47,48
The role of functional foods
Nutrition is truly functional during pregnancy and lactation, because it exerts prenatal and early postnatal influences on the developing baby: maternal nutrition affects the intra-uterine development of the baby and determines the quality of the breast milk needed to support adequate growth and gut-flora composition.
The more commonly used approach to functional foods involves designed foods in which ingredients have been added or removed. Only the former category will be considered here. Different types of designed foods are classified as functional foods: prebiotics and probiotics, vitamins and minerals, bioactive molecules, and fatty acids.
Probiotics are life microbial food ingredients (bacteria, yeasts, microalgae) that are beneficial for health.49 They are mostly lactobacilli and Bifidobacteria and either used as freeze-dried cultures (in capsules) or to prepare fermented dairy products (yoghurt or sour milk).
Probiotics added to food products must meet several criteria such as a beneficial effect on health, survival during transit through the gastrointestinal tract, adhesion (permanently or temporarily) to the intestinal epithelial cell lining, production of antimicrobial substances toward pathogens or stabilisation of the intestinal microflora. Over-the-counter supplements, however, may not fulfil these criteria and may not even survive in the gastrointestinal tract.50
With particular relevance for the subject on hand are several trials with either pregnant women, lactating mothers and their babies, or with children, that have demonstrated several beneficial effects of probiotics. These include the maturation and health of the intestinal tract and the immune system, the reduction of lactose intolerance and allergy prevalence, the reduction of the risk of microorganism-induced diarrhoea, or the enhancement of nutrient bioavailability.51,52,53,54,55,56,57
Not only are probiotics therefore promising functional foods for pregnant women and infants, but they can be considered for prophylactic as well as therapeutic uses. Prophylactic use of probiotics for women during the last trimester of pregnancy and through childbirth, for instance, permanently colonised the gastrointestinal tracts of their infants. 58 It is not yet known whether the immune-boosting properties of these probiotics require periodic pulse dosing or continuous administration. 58
Probiotics given to pregnant and lactating mothers increased the immuno-protective potential of breast milk and reduced the incidence of atopic eczema during the first two years of life in their children.54
Another study showed that in addition to allergy occurrence, the number of infections and the need for antibiotics due to preventive probiotic treatment after birth were reduced even ten years later.59
Preventive feeding of fermented milk also increased the absorption of iron due to the liberation of lactic acid and other organic acids during fermentation.60 The authors even suggested that consumption of fermented milk during meals might also have a positive effect on the absorption of iron from other foods. Based on such findings and the fact that even temporary colonization of a baby's intestines with probiotic bacteria prevents colonization with less beneficial bacteria, probiotic supplementation of milk formula has been proposed.
Prebiotic foods are non-digestible food ingredients that beneficially affect the host by selectively stimulating the growth and/or the activity of one or a limited number of bacteria in the colon.49
Prebiotic oligosaccharides from different origins have been used as ingredients in functional foods. They may be inulin; lactulose; fructo-, galacto-, isomalto- or xylo-oligosaccharides.
According to their chemical nature they support higher populations of individual bacterial species in the gut flora.61 The largest increase in lactobacilli was seen with xylo-oligosaccharides and lactulose. Although fructo-oligosaccharides promoted a large increase in lactobacilli, they also supported higher populations of streptococci than did galacto-oligosaccharides. The latter supported higher populations of Bifidobacteria and higher levels of lactate than fructo-oligosaccharides.62 Lactulose and xylo- and galacto-oligosaccharides thus stimulate the growth of bacteria found in the colon of breast-fed infants. Formula-fed infants, on the other hand, have a more diverse and adult microflora and tend to suffer more from microbial infections than breast-fed infants.62
This means that lactulose, xylo- and galacto-oligosaccharides are the prebiotic oligosaccharides of choice for functional foods aimed at infants. Supplementing milk formula with these oligosaccharides should therefore circumvent the problem of aberrant colon colonization in formula-fed infants. However, prebiotic functional foods will be effective only where there is a real need, since responses to prebiotics depend on the numbers of bacteria colonizing the colon. Individuals with low Bifidobacterial counts displayed much higher responses to prebiotics than individuals with higher bacterial counts.62
Prebiotics positively affect the absorption of various minerals as well as mineral contents in bones.63,64 The risk of osteoporosis is higher in formula-fed children than in breast-fed children born at term,65 even though milk formula has a higher calcium content than breast milk.66 Prebiotic supplementation of milk formula might thus help reduce the risk of osteoporosis in formula-fed children born at term.
This finding does not apply, however, to children born pre-term, where the source of milk does not seem to influence bone mass later on.67 Nevertheless, it might be worthwhile to follow these term and pre-term children into adult age to check for possible long-term protection against osteoporosis due to early prebiotic supplementation.
Future trends
There is a clear need for identifying biomarkers of nutritional status and developing standardised bioassays to estimate this nutritional status as well as the nutritional requirements at different periods of life. More research is required on the metabolic fate of essential nutrients and on the interactions between individual nutrients. Equally lacking are data on nutrient impact, on gene expression and body functions, and on intellectual or physical performance. This is particularly the case for pregnant and lactating women and their infants.
Most of the increase in energy intake in US children and adolescents is already accounted for by snacks and/or evening meals,68 fast-food meals providing most of the total and saturated fat consumed.69 With regard to the nutrition of pregnant women and children, this will increase the risk of nutritional imbalances due to skipped meals and uncontrolled food consumption in these particularly vulnerable population groups.
Luise Kalbe, PhD, Brigitte Reusens, PhD, and Professor Claude Remacle are in the cellular biology laboratory at Universite Catholique de Louvain, Belgium.
Excerpted from Functional Foods, Ageing and Degenerative Disease, C Remacle and B Reusens, editor. ISBN 1 85573 725 6. Published by Woodhead Publishing Ltd, England. www.woodheadpublishing.com
Respond: [email protected]
References
1. Barker DJ. Mothers, babies and disease in later life. London BMJ. 1994.
2. Forsdahl A. Are poor living conditions in childhood and adolescence an important factor for arteriosclerotic heart disease. Br J Prev Soc Med 1977;31:91-5.
3. Barker DJ, Osmond C. Infant mortality, childhood nutrition, and ischaemic heart disease in England and Wales. Lancet 1986;1:1077-81.
4. Barker DJ, et al. Weight in infants and death from heart disease. Lancet 1989;2:577-80.
5. Leon D. Fetal growth and adult disease. Eur J Clin Nutr 1998;52;(suppl 1):S72-S82.
6. Forsen T, et al. Growth in utero and during childhood among women who develop coronary heart disease. Brit Med J 1999;319:1403-7.
7. Rich-Edwards JW, et al. Birthweight and risk of cardiovascular disease in a cohort of women followed up since 1976. Brit Med J 1997;315:396-400.
8. Stein CE, et al. Fetal growth and coronary heart disease in South India. Lancet 1996;348:1269-73.
9. Hales CN, et al. Fetal and infant growth and impaired glucose tolerance at age 64. Br Med J 1991;303:1019-22.
10. Barker DJ, et al. Type 2 diabetes mellitus, hypertension and hyperlipidaemia (syndrome X): relation to reduced fetal growth. Diabetologia 1993;36;62-7.
11. Girard J, et al. Adaptations of glucose and fatty acid metabolism during perinatal period and suckling-weaning transition. Physiol Rev 1992;72:507-62.
12. Catalano PM, et al. Maternal factors that determine neonatal size and body fat. Curr Diab Rep 2001;1:71-7.
13. Clapp JF. Maternal carbohydrate intake and pregnancy outcome. Proc Nutr Soc 2002;61:45-50.
14. Godfrey K, et al. Maternal nutrition in early and late pregnancy in relation to placental and foetal growth. Br Med J 1996;312:410-4.
15. Campbell DM, et al. Diet in pregnancy and the offspring's blood pressure 40 years later. Br J Obst Gynecol 1996;103:273-80.
16. Al MD, et al. Maternal essential fatty acid patterns during normal pregnancy and their relationship with the essential fatty acid status. Br J Nutr 1995;74:55-68.
17. Herrera E. Implications of dietary fatty acids during pregnancy on placental, fetal and postnatal development. Placenta (Trophoblast Res) 2002;23(16):S9-S19.
18. Koletzko B. Fats for brains. Eur J Clin Nutr 1992;46 (Suppl. 1):S51-S62.
19. Secher NJ, Olsen SF. Fish oil and preeclampsia. Br J Obst Gynecol 1990;97:1077-9.
20. Olsen SF, et al. Intake of marine fat, rich in (n-3) polyunsaturated fatty acids, may increase birth weight by prolonging gestation. Lancet 1986;2:367-9.
21. Olsen SF, et al. Randomized controlled trial of the effect of fish-oil supplementation on pregnancy duration. Lancet 1992;339:1003-7.
22. Haggarty P, et al. Effect of maternal polyunsaturated fatty acid concentration on transport by the human placenta. Biol Neonate 1999;75:350-9.
23. Picciano MF. Pregnancy and lactation: physiological adjustments nutritional requirements and the role of supplements. J Nutr 2003;133:1997S-2002S.
24. Duggleby SC, Jackson AA. Protein amino acid and nitrogen metabolism during pregnancy: how might the mother meet the needs of her fetus? Curr Opin Clin Nutr Metab Care 2002;5:503-9.
25. Bung P, et al. Idiopathic infertility and sterility: does folic acid play a key role? Journal fur Fertilitat und Reproduktion 1995;2:21-4.
26. Pietrzik K, et al. Folate status and pregnancy outcome. Ann NY Acad Sci 1992;669:371-3.
27. George L, et al. Plasma folate levels and risk for spontaneous abortions. JAMA 2002;288:1867-73.
28. Bailey LB, et al. Folic acid supplements and fortification affects the risk for neural tube defects, vascular disease and cancer. J Nutr 2003;133:1961S-1968S.
29. Hague WM. Homocysteine and pregnancy. Best Pract Res Clin Obstet Gynaecol 2003;17:459-69.
30. Lonn E, et al. Heart Outcomes Prevention Evaluation (HOPE) 2 Investigators. Homocysteine lowering with folic acid and B vitamins in vascular disease. N Engl J Med 2006 Apr 13;354(15):1567-77.
31. Zeisel SH. Choline: needed for normal development of memory. J Am Coll Nutr 2000;19:528S-531S.
32. Zeisel SH, et al. Pregnancy and lactation are associated with diminished concentrations of choline and its metabolites in rat liver. J Nutr 1995;125:3049-54.
33. Koldovsky O, Thornburg W. Hormones in human milk: a review. J Pediatr Gastroenterol Nutr 1987;6:172-96.
34. Koldovsky O, Strbak V. Hormones and growth factors in human milk. In Handbook of milk composition, pp 428-36 (RG Jensen, ed.) San Diego, CA:Academic Press. 1995.
35. Kelley DS, Erickson KL. Modulation of body composition and immune cell functions by conjugated linoleic acid in humans and animal models: benefits vs. risks. Lipids 2003;38:377-86.
36. Allen LH. Iron supplements: Scientific issues concerning efficacy and implications for research and programs. J Nutr 2002;132:813S-819S.
37. Preziosi P, et al. Effect of iron supplementation on the iron status of pregnant women: consequences for newborns. Am J Clin Nutr 1997;66:1178-82.
38. Werler MM, et al. Prepregnant weight in relation to risk of neural tube defects. JAMA 1996;275:1089-92.
39. Prentice A, Goldberg G. Maternal obesity increases congenital malformations. Nutr Rev 1996;54:146-50.
40. Rassin DK, et al. Taurine and other amino acid in milk of man and other mammals. Early Hum Dev 1978;2:1-13.
41. Gaull GE, et al. Milk protein quantity and quality in low-birth weight infants: Effects of sulfur amino acids in plasma and urine. J Pediatr 1977;90:348-55.
42. Gaull GE, et al. Brain and nutrition: the role of taurine. Prog Clin Biol Res 1982;91:349-55.
43. Galli C, Marangoni F. Recent advances in the biology of n-6 fatty acids. Nutr 1997;13:978-85.
44. Simopoulos AP. Omega-3 fatty acids in health and disease and in growth and development. Am J Clin Nutr 1991;54:438-63.
45. Simopoulos AP. Symposium: role of poultry products in enriching the human diet with n-3 PUFA. Poultry Sci 2000;79:961-70.
46. Helland IB, et al. Maternal supplementation with very long chain n-3 fatty acids during pregnancy and lactation augments children's IQ at 4 years of age. Pediatrics 2003;111:e39-e44.
47. Villar J, et al. Nutritional interventions during pregnancy for prevention or treatment of maternal morbidity and preterm delivery: an overview of randomized controlled trials. J Nutr 2003;133:1606S-1625S.
48. Roberts JM, et al. Nutrient involvement in preeclampsia. J Nutr 2003;133:1684S-1692S.
49. Roberfroid MB. Prebiotics and probiotics: are they functional foods? Am J Clin Nutr 2000;71:(Suppl)1682S-1687S.
50. Kopp-Hoolihan L. Prophylactic and therapeutic uses of probiotics: a review. J Am Diet Assoc 2001;101:229-38.
51. Schiffrin EJ, Blum S. Interactions between the microbiota and the intestinal mucosa. Eur J Clin Nutr 2002;56(Suppl. 3):S60-S64.
52. Cunningham-Rundles S, et al. Probiotics and immune response. Am J Gastroenterol 2000;95(Suppl):S22-S25.
53. Shermak MA, et al. Effect of yoghurt on symptoms and kinetics of hydrogen production in lactose-malabsorbing children. Am J Clin Nutr 1995;62:1003-6.
54. Rautava S, et al. Probiotics during pregnancy and breast-feeding might confer immunomodulatory protection against atopic disease in the infant. J Allergy Clin Immunol 2002;109:119-21.
55. Saavedra JM, et al. Feeding of Bifidobacterium bifidum and Streptococcus thermophilus to infants in hospital for prevention of diarrhoea and shedding of rotavirus. Lancet 1994;344:1046-9.
56. Guandalini S, Dincer AP. Nutritional management in diarrheal disease. Baillieares Clin Gastroenterol 1998;12:697-717.
57. Branca F, Rossi L, The role of fermented milk in complementary feeding of young children. Eur J Clin Nutr 2002;56(Suppl 4):S16-S20.
58. Vanderhoof JA. Probiotics: future directions. Am J Clin Nutr 2001;73:(Suppl)1152S-1155S.
59. Lodinova-Zadnikova R, et al. Oral administration of probiotic Escherichia coli after birth reduces frequency of allergies and repeated infections later in life (after 10 and 20 years). Int Arch Allergy Immunol 2003;131:209-11.
60. Branca F, Rossi L. The role of fermented milk in complementary feeding of young children: lessons from transition countries. Eur J Clin Nutr 2002;56(Suppl 4):S16-S20.
61. Rastall RA, Maitin V. Prebiotics and synbiotics: towards the next generation. Curr Op Biotechnol 2002;13:490-6.
62. Harmsen HJM, et al. Analysis of intestinal flora development in breast-fed and formula-fed infants by using molecular identification and detection methods. J Pediatr Gastroenterol 2000;30:61-7.
63. Scholz-Ahrens KE, et al. Effects of probiotics on mineral metabolism. Am J Clin Nutr 2001;73:459S-464S.
64. Roberfroid MB, et al. Dietary chicory inulin increases whole-body bone mineral density in growing male rats. J Nutr 2002;132:3599-3602.
65. Jones G, et al. Breastfeeding in early life and bone mass in prepubertal children: a longitudinal study. Osteoporos Int 2000;11:146-52.
66. Bosscher D, et al. Availabilities of calcium, iron, and zinc from dairy infant formulas is affected by soluble dietary fibers and modified starch fractions. Nutr 2003;19:641-5.
67. Fewtrell MS, et al. Bone mineralization and turnover in preterm infants at 8-12 years of age. J Bone Miner Res 1999;14:810-20.
68. Gleason P, Suiter CW. Changes in children's diets: 1989-1991 to 1994-1996. US Department of Agriculture, Food and Nutrition Service. January. Available at www.fns.usda.gov/oane/MENU/Published/CNP/CNP.htm
69. Lin B, et al. America's children's diets not making the grade. Food Rev 2001;24:8-17.
You May Also Like