thumbnail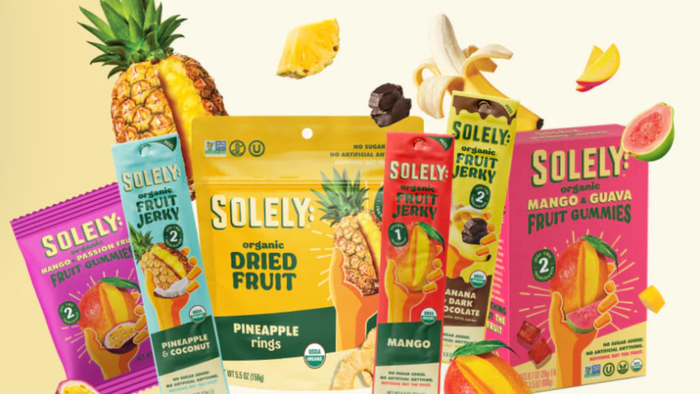
Industry Insights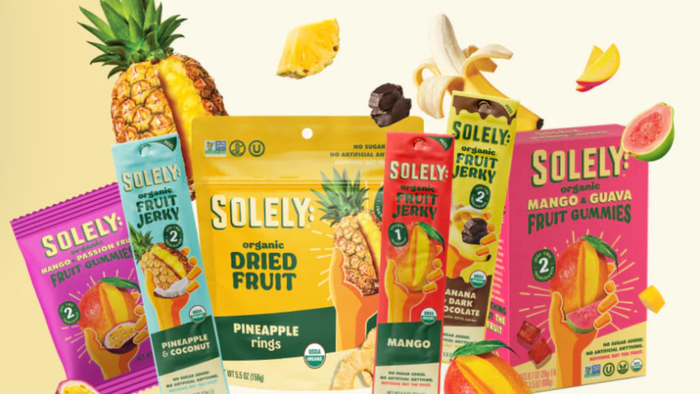
Crafting a sweet future: How natural solutions are redefining snackingCrafting a sweet future: How natural solutions are redefining snacking
Simón Sacal, the CEO and founder of Solely, talks about why committing to reducing sugar in CPG is vital for both people's health and the planet.
Subscribe and receive the latest updates on trends, data, events and more.
Join 57,000+ members of the natural products community.